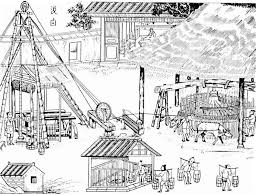
In this issue of our newsletter we will discuss what is considered to be the modern history of lubrication. Early machines were relatively simple and worked satisfactorily using crude oil as a lubricant. Most industrial plants kept a single drum of oil to lubricate all their machines. During the First Industrial Revolution (1760 to 1840) the development of larger machines with tighter specifications which had to run at greater speeds for mass production, triggered the search for more sophisticated and specialised lubricants.
It is impossible to discuss all the highlights in the evolution of modern lubrication in a space like this, but we consider the following milestones worth mentioning:
1848 – Scottish chemical engineer James Young (founder of the first commercial oil works in the world and father of the petrochemical industry) discovered natural petroleum seepage in a Derbyshire colliery. From this he distilled a light thin oil for use as lamp oil, and at the same time obtaining a thicker oil suitable for lubricating machinery.
1859 – Edwin L. Drake struck oil at Titusville in America. His oil well created a new way to supply petroleum-based oil products, which accelerated the move toward the use of mineral oil in America.
1866 – Dr John Ellis, founder of the Continuous Oil Refining Company in Binghamton, USA, developed an all-mineral, high viscosity lubricant for steam engines – previously using inefficient combinations of petroleum and animal or vegetable fats. His breakthrough oil worked very effectively at high temperatures. This meant no more gummed valves, corroded cylinders or leaking seals.
1872 – Elijah McCoy, a Canadian-born American inventor and engineer, developed an automatic lubricator that was used to apply oil through a drip cup to locomotive and ship steam engines. Railroad engineers insisted on McCoy lubrication systems when buying new machines and would take nothing less than what became known as “the real McCoy”.
1885 – German engineer Karl Benz produced a petrol-powered automobile. This is also considered to be the first “production” vehicle as Benz made several other identical reproductions. The vehicle was powered by a single cylinder four-stroke engine.
1908 – Henry Ford introduced The Ford Model T car on October 1, 1908. During 1913 the Model T became the first automobile to be mass-produced on a moving assembly line. By 1927, Ford had produced over 15,000,000 Model T cars.
1920 – As the demand for automobiles grew, so did the demand for better lubricating oils. Lubricant manufacturers started extracting base oils under vacuum from crude oil to improve the performance of their lubricants. Vacuum distillation is still used today in modern oil refineries.
1923 – The Society of Automotive Engineers (SAE) classified engine oils by viscosity i.e. light, medium and heavy. At the time engine oils contained no additives and had to be replaced every 800 to 1000 miles.
1930s Up to 1930 there were no common standards to regulate the performance of motor oils and every vehicle manufacturer had their own set of requirements for lubricants. Vehicle manufacturers realized that there was a need for fixed standards for lubricant performance so that vehicles could be sold anywhere in the world without major modifications or embarrassing failures. The American Petroleum Institute (API) took on the task of setting common standards for engine oil. Their first attempt described three oil categories: Regular (straight mineral oil); Premium (mineral oil with oxidation inhibitors) and Heavy Duty (mineral oil with oxidation inhibitors plus detergents/dispersants). Zinc dialkyl phosphate additives were developed to provide rust and corrosion protection. The antiwear properties of zinc compounds were only recognised years later.
1940s – World War II accelerated the development of lubricant technology and the use of molybdenum disulphide as a solid lubricant dates back to the early days of the war. America and Germany started using ester based synthetic oils in fighter plane engines. Polyalphaolefin (PAO) synthetic oils were developed in the USA and their initial use was in steam turbines. Possibly the first ever use of synthetics for automotive applications was in tank engines. Sherman tanks operating in the intense heat of the Sahara Desert were enormously stressed. The engines fried their oil and seized solid. The new PAO lubricants were tried out very successfully in the tank engines. In 1947, after the war, the API changed their Engine Oil Classification System to the format still used today.
1950s – It was only during the nineteen-fifties that the antiwear properties of zinc chemistry were fully understood and it became an integral part of many oil formulations. To this day zinc dialkyl phosphates remain the backbone of antiwear additive technology. Solvent oil refining emerged and replaced traditional petroleum distillates owing to their improved lubricant properties. This eventually led to the introduction of API Group 1 lubricating base oils. The world’s first successful multigrade engine oil was launched in 1953. Fire Resistant Hydraulic Fluids were invented in 1958. The first Jet Engine Oil based on polyol-esters were developed and patented in New Jersey, USA. This decade also saw the development of superior grease types, i.e. urea and aluminium.
1960s – Food grade lubricants were introduced and used since the early 1960s. The Jost Report in 1966 (click to follow link) led by Dr Peter Jost, included the first mention of the term “tribology”.
1970s – The first 100% synthetic di-ester based engine oil passed the API sequence tests and received API qualification in 1972. The following year the first PAO based synthetic engine oil was launched commercially.
1980s – A low-wear tractor hydraulic fluid (THF/UTTO) with satisfactory wet brake performance in a wide variety of tractors was introduced in 1980. The development and design of American and European engines and their appetite for lubricants departed from each other and the CCMC (Committee of Common Market Automobile Constructors) promulgated oil specifications for European automotive engines. 1984 saw the introduction of all-hydroprocessed base oils in the USA. This process combined catalytic dewaxing with hydrocracking and hydrofinishing, which set a new standard for performance (API Group II) in the lubricating base oil industry.
1990s – Germany pioneered SAE 0W-60 engine oil during 1992. The European Automobile Manufacturers’ Association (ACEA) Oil Sequences were introduced in 1996 when they superseded the outdated CCMC
Sequences previously used to define European petrol and diesel engine oil performance. A revolutionary base oil dewaxing process (hydroisomerization) was commercialized in Richmond, California. Hydroisomerization converts petroleum wax to very high-quality base oil. This represented a major improvement over Group I and II base stocks and allowed the introduction of superior quality Group III base oils. Although these oils were (and still are) produced from mineral feed stocks,
Group III oils closely mirrored the characteristics of PAO synthetic base oils. In fact, during 1999 the National Advertising Division (NAD) in America ruled that hydroisomerized Group III base oil can be classified as ‘synthetic oil’.
2000 and Beyond
The lubricant evolution continues as increasingly advanced products are developed to meet the rising demands of modern machinery for better productivity, performance reliability, energy efficiency and environmental responsibility. To meet these requirements the trend is towards lower viscosity oil grades, alternative additive technologies and base oils with higher purity and lower volatility. Many modern engines are now calling for SAE 5W-30 and even SAE 0W-20. In fact the SAE (Society of Automotive Engineers) has recently promulgated a new SAE 16 grade as a lighter alternative to SAE 20 engine oil grade. In the interest of the environment, focus has shifted to low ash oil additives with little or no metallic compounds. New Group III base oils have been developed that can actually outperform a full synthetic PAO in several areas important to lubrication, such as additive solubility, lubricity and antiwear performance. Group III base oils can now also rival PAO stocks in pour point, viscosity index and oxidation stability and can be manufactured in volumes and at a price unachievable by PAOs.
With the support of Q8Oils, Blue Chip Lubricants are at the forefront of new product development. Our vast experience, well equipped laboratory and modern production facilities ensure that you always receive a tailor-made solution for your specific lubrication requirements. To learn more please contact us today by visiting https://www.bcl.co.za